Best use of renewable hydrogenVarious applications, carbon intensity of hydrogen, and substitute products
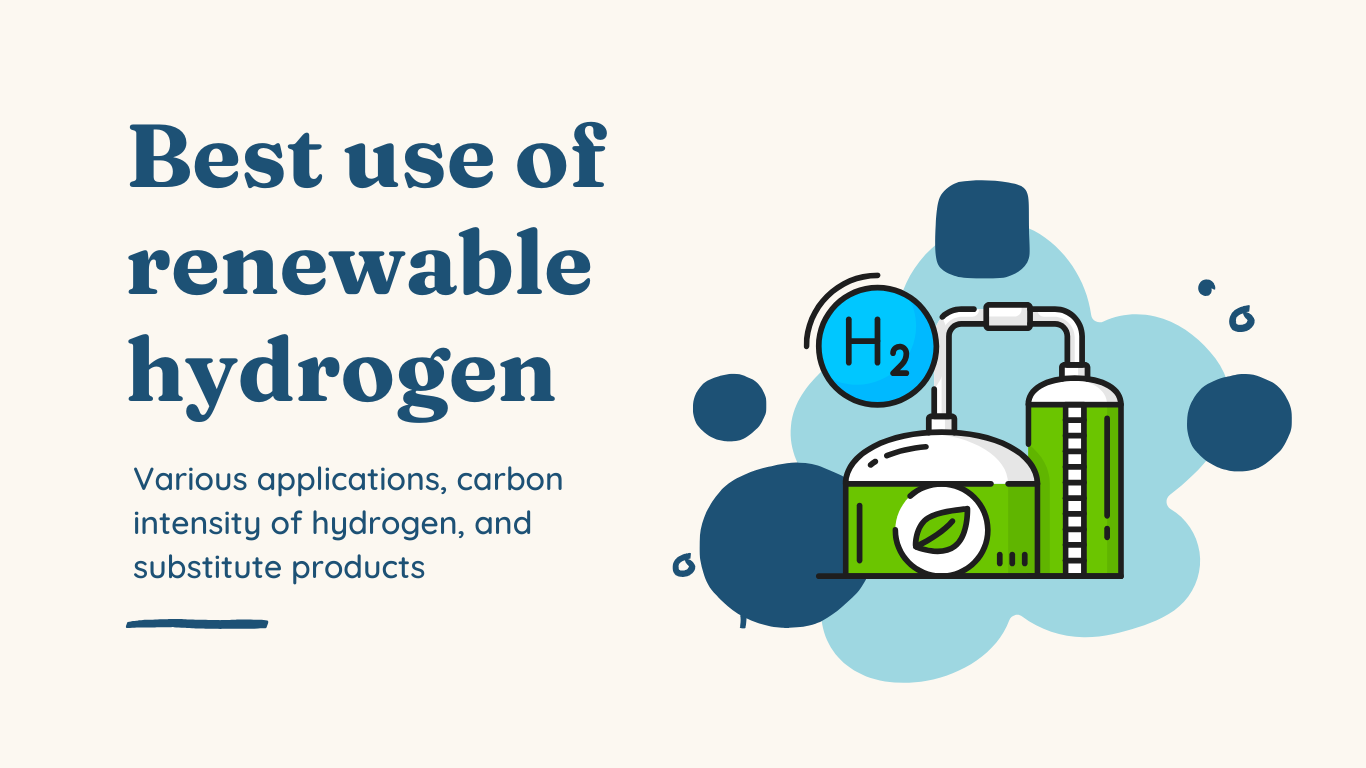
The U.S. Inflation Reduction Act (IRA) incentivizes the decarbonization of a wide range of energy sectors, including hydrogen. This article analyzes various hydrogen applications and their life cycle GHG emissions using the 45VH2-GREET model to identify best use case applications for green hydrogen and provides insights for greater uptake.
Green hydrogen options
Approximately 10 million tonnes of hydrogen are currently utilized in various industrial applications in the United States, which is mostly produced from natural gas (Shearman, 2021). The Inflation Reduction Act (IRA), specifically Section 45V concerning the clean hydrogen production credit, supports the expansion of green hydrogen applications through tax credits that increase based on reductions in carbon intensity (CI). Green hydrogen refers to the production of hydrogen via water electrolysis and low CI electric power. The goals of the IRA and the U.S. National Clean Hydrogen Strategy and Roadmap are to expand the use of green hydrogen to reduce GHG emissions and create new jobs in the sector (DOE, 2023).
While these frameworks are sector and technology agnostic relative to green hydrogen applications, new technologies will contribute the most substantive GHG reductions. Figure 1 shows current global uses for hydrogen. Oil refining is the leading use, with most of it produced from natural gas. Other uses include ammonia production, methanol, steel manufacturing, and heat and chemical applications. In each application, green hydrogen could displace the fossil incumbent. The overall impact of green hydrogen in reducing GHG emissions will depend not only on its carbon intensity but also on how it is deployed.
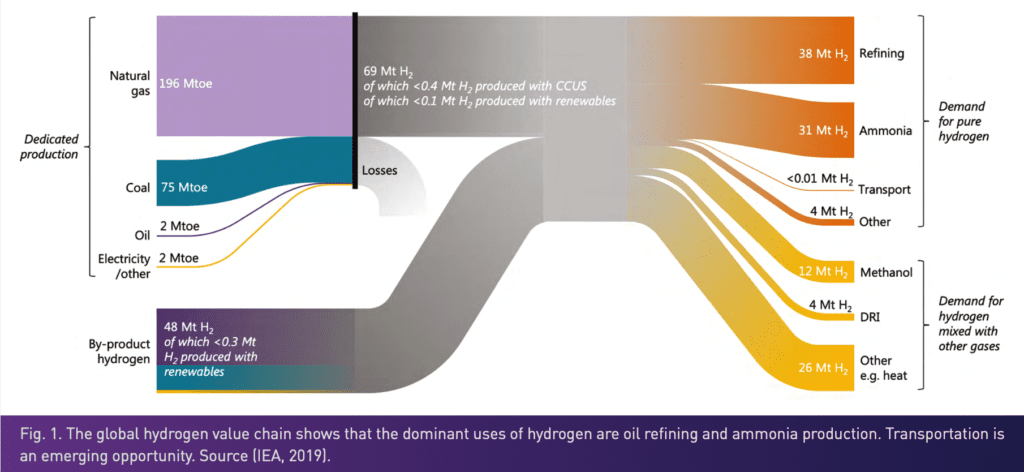
The three pillars under the IRA
Under Section 45V of the IRA, three pillars form the framework for green hydrogen to qualify for tax credits: the power generation would be time coincident, from new resources, and regionally connected (Federal Register, 2023). These requirements are intended to assure that low CI electric power is not diverted from existing customers to green hydrogen applications, yielding zero net environmental benefit.
Achieving the lowest GHG score and maximum benefit under the IRA requires low CI electric power. The power will need to have a CI below 8 g CO2e/kWh in order to achieve the IRA threshold of 0.45 kg CO2e/kg of H2, which is feasible with renewable or nuclear power. In the case of fossil fuel generation, the CI is well above 400 g CO2e/kWh; so even a small fraction of fossil power would exclude hydrogen from achieving the lowest IRA threshold value. The Internal Revenue Service (IRS) mandates that hydrogen eligible for 45V credits must not be used inefficiently while supporting emerging applications (IRS, 2023).
The IRA guidance in Section 45V is sector and technology agnostic even though end use applications have different impacts on overall CI displacement. Natural gas-based pathways with CO2 capture (blue hydrogen) could potentially qualify for credits under Section 45V. However, the three pillars will also apply to renewable power used to operate autothermal reformers. Proposed limitations on the IRA could also impact the incentive value for hydrogen projects at oil refineries (Martin, 2024). While reducing emissions from oil refineries will displace current hydrogen production emissions, continuing to refine crude oil is also an indirect impact of clean production.
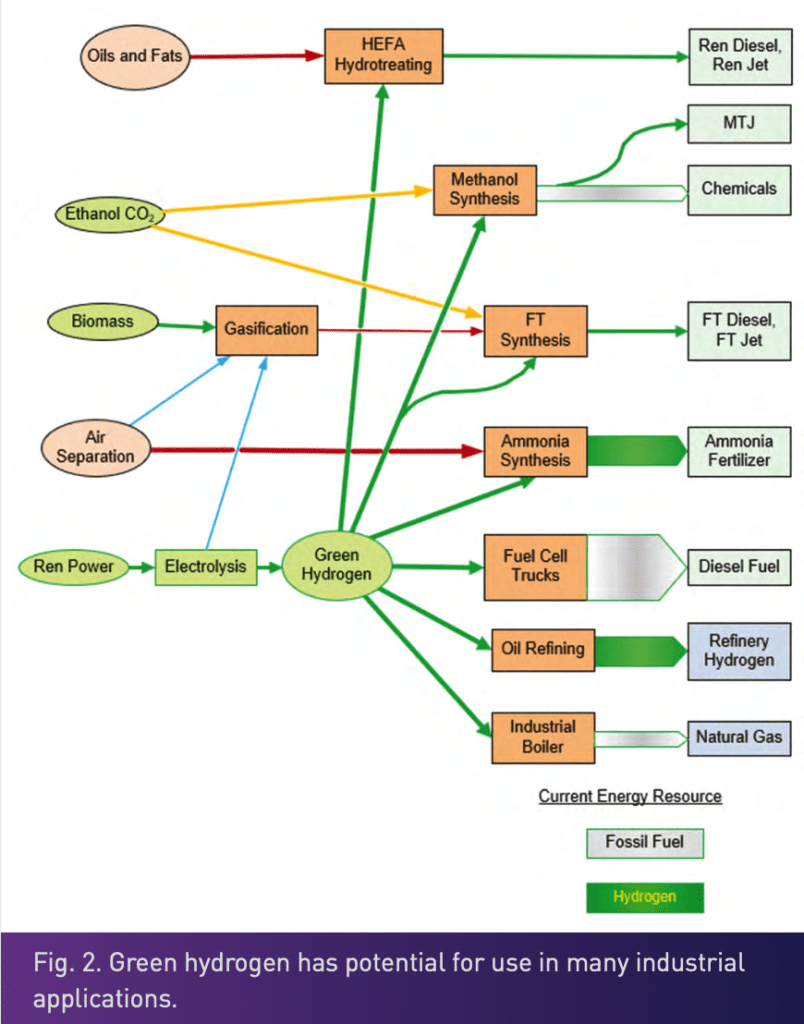
Figure 2 shows a range of potential green hydrogen applications and some of the displaced products. Several new applications have additional environmental benefits due to displacing more emissions or advancing technology in new sectors. For example, expanding renewable diesel facilities will rely on new sources of natural gas-based hydrogen or the use of renewable naphtha, which could otherwise be used as a chemical feedstock to produce low GHG materials. eFuels, such as methanol-to-jet (MTJ) or Fischer Tropsch fuels derived from green hydrogen and waste CO2, provide an opportunity to decarbonize the aviation sector. Ammonia is a key component to agriculture and it has a relatively high carbon intensity. The use of hydrogen in fuel cell vehicles will result in zero- emission transportation and displace twice the amount of diesel energy in trucking applications.
An additional benefit of the production of green hydrogen is the co-product oxygen. The oxygen co-product from electrolyzers or air separation units could be integrated with biomass gasification. This integration implies a potential synergy where the oxygen can be utilized to contribute to more sustainable industrial practices. Green hydrogen used as a boiler fuel could reduce the GHG footprint of industrial processes and even reduce the carbon intensity of natural gas-intensive fuels such as corn ethanol.
Carbon intensity of hydrogen and substitute products
Under the IRA, the U.S. Department of Treasury adopted a specific version of the GREET model, 45VH2-GREET, to determine GHG reductions for Section 45V. The model is used to calculate the life cycle GHG emissions from hydrogen production under a well-to-gate system boundary. GHG emissions from green hydrogen depend on the displaced incumbent fossil fuel. In the case of refinery hydrogen and process heat, green hydrogen provides a 1:1 displacement of natural gas steam methane reforming (SMR) hydrogen on an energy basis. For ammonia, methanol, and on-road transportation fuels, the displacement ratio is pathway-dependent. In fuel cell vehicles, one kilogram of hydrogen displaces approximately double the energy content of petroleum diesel.
Table 1 shows potential applications for green hydrogen and the displaced product. The CI of displaced products varies between 69.1 and 126.9 g/MJ of hydrogen. The hydrogen use rate per unit of product, along with CI, facilitates the calculation of GHG reductions per kilogram of green hydrogen. Considering this calculation, the GHG benefit per kilogram of green hydrogen used for ammonia, methanol, and trucking suggests they are the best sectors to achieve the GHG abatement goals of the IRA.
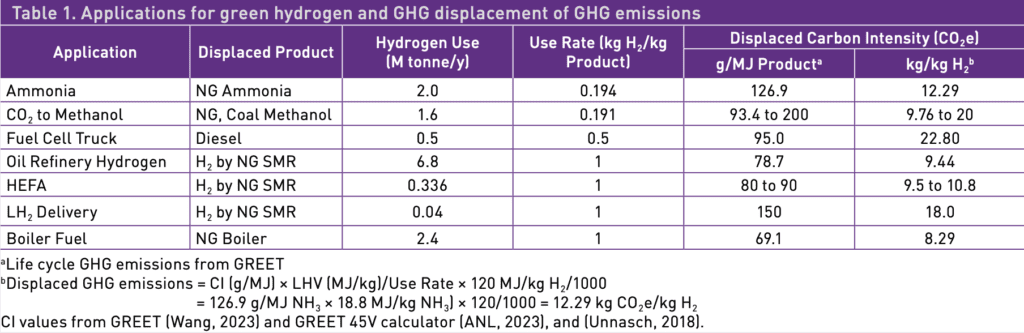
Best uses of green hydrogen
Any use that displaces a fossil fuel will reduce GHG emissions; however, applications such as ammonia, fuel cell vehicles, and LH2 delivery provide the greatest emission reductions per kilogram. Figure 3 demonstrates the displaced GHG emissions from green hydrogen uptake, based on the end-use application. The largest-diameter circle corresponds to greater emission reductions. while the smaller blue circles represent established uses where green hydrogen will have less of an impact.
A few components from the preliminary guidance on the requirements for Section 45V of the IRA are worthy of comment to ensure the best uses of green hydrogen achieve even greater uptake. These include the requirements for the three pillars of renewable power, matching choices of feedstocks, and considerations for indirect effects.
Achieving time-of-use additionality and regional location requirements for renewable power is an ideal goal pursued by many developers. A current challenge, however, is that such requirements are evolving, and developers will have difficulty in procuring renewable power in the near term due to current capacity constraints. A transition period, similar to the one allowed under the European Union rules for renewable fuels of non-biological origin (RFNBOs) would be appropriate (EU Commission, 2023). The time horizon for EU producers is through 2045, creating an unlevel playing field for U.S. producers constrained to an immediate requirement.
Matching also poses potential challenges as end users of green hydrogen may be unable to adjust their production processes to an intermittent flow of hydrogen based on available renewable energy.
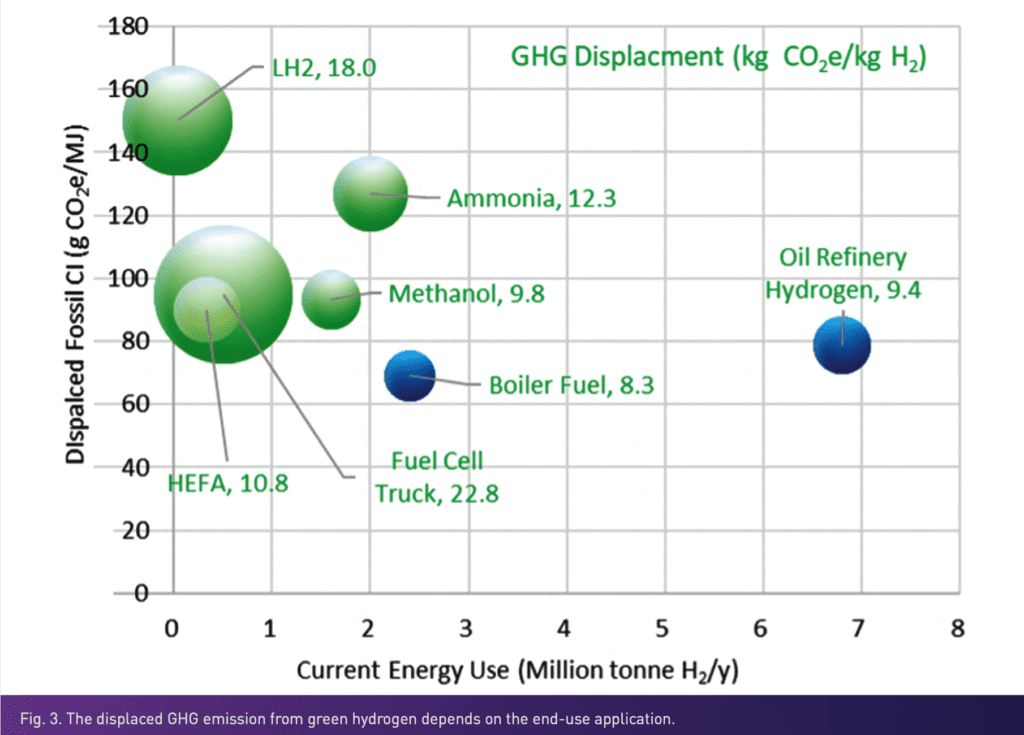
Hydrogen use technologies such as liquefaction, ammonia, and methanol production do not readily accommodate on/off operation considering the cold temperature for liquefaction or high temperatures conversion reactions. These factors are in addition to challenges related to availability of funding, ability to secure proper permitting, and in securing offtake agreements. Combined, these challenges lead to project announcements across the sector that fail to get underway, even while accounting for available tax credits.
Many proposed hydrogen technologies provide significant environmental benefits above and beyond simply displacing fossil hydrogen from natural gas (EIA, 2023). For example, hydrogen powering fuel cell vehicles displaces double the volume of diesel on an energy basis, signifying a notable indirect effect in end-use applications. Prioritizing applications with the most substantial environmental benefit would support the intended goals of the IRA and the strategic green hydrogen roadmap. Storing renewable power will also be costly in the near term. Therefore, the best pathway to success with green hydrogen may lie in a combination of relief on three pillars with technologies that have the largest impact on GHG reductions.
Note
* 8 g CO2e/kWh ” 55 kWh/kg H2 = 0.44 kg CO2e/kg H2.
References
ANL. (2023). Guidelines to Determine Well-to-Gate Greenhouse Gas (GHG) Emissions of Hydrogen Production Pathways using 45VH2-GREET 2023.
Chao, J. (2017, October 6). Berkeley Lab.
DOE. (2023). U.S. National Clean Hydrogen Strategy and Roadmap.
EIA. (2019, February 21). New methanol plants expected to increase industrial natural gas use through 2020.
EIA. (2021, March 21). Natural Gas Weekly Update. EIA. (2023, April 28). Natural Gas Explained.
EU Commission. (2023, February 13). Commission Sets Out Rules for Renewable Hydrogen.
Federal Register. (2023, December 26).
IEA. (2019). The Future of Hydrogen: Seizing today’s opportunities.
IRS. (2023, December 26).
Le PA, T. V. (2023 Sep 25). The current status of hydrogen energy: an overview. RSC Adv 13.40: 28262–28287.
Martin, P. (2024, February 23). Hydrogen Insight.
Sustainability 15.2 (2023): 1623.
Hydrogen’s present and future in the US energy sector. (2021, October 7). A&O Shearman.
Journal of CO2 Utilization 68 (2023): 102345.
Unnasch, S. (2018). GHG Analysis of Kalama Manufacturing and Marine Export Facility. Life Cycle Associates Report LCA.6132.185.2018, prepared for Northwest Innovation Works.
Wakelin, S., & Beets, P. (2021). Emission factors for managed and unmanaged Grassland with Woody Biomass. Ministry for the Environment.
Wang, M. (2023). Summary of Expansions and Updates in R&D GREET® 2023. ANL.
About us
Life Cycle Associates is a trusted partner for clients worldwide, assisting in emissions reduction and environmental impact mitigation. Our expertise lies in life cycle greenhouse gas emission analysis of fuel and energy production pathways, with extensive use of models such as GREET, IRA 45V, and GTAP.
We offer services that include the modification of the California GREET model, development of initial pathway documents for the LCFS, support for fuel pathways under the LCFS, RFS2, and EU Renewable Energy Directive (RED), as well as determining the applicability of Inflation Reduction Act (IRA) provisions.
A significant portion of our work in biofuels and other alternative fuels concerns the evaluation of new fuel production technologies, their energy balance, and economics.
This article was originally published in Hydrogen Tech World (pages 26-30).
By Stefan Unnasch, Managing Director; Brian D. Healy, Chief Economist; Abhishek Kumar, Environmental Scientist; and Kathleen Dailey, Business Development Engineer, Life Cycle Associates, LLC.